Project 1.1 – Thermochemical Conversion of Biomass to H2 (Julia Valla)
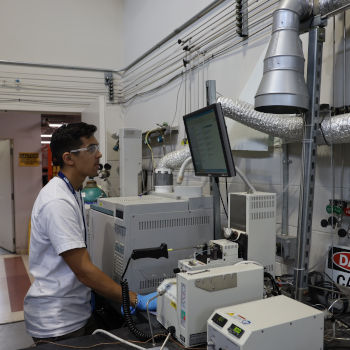
Background: H2 and H2 rich gases can be produced from biomass by thermochemical processes, such as pyrolysis or gasification. The efficiency of the process depends on feedstock type (residual agricultural waste, animal waste, plastics, etc.), catalyst employed and operating conditions. Dr. Valla’s research interests lie in the field of conversion of lignocellulosic and waste resources into energy and fuels.
Objectives: During the Year 1 of this project the participant students will develop models using Aspen Plus® to simulate the gasification and pyrolysis processes of three waste resources: miscanthus, food waste and plastic bottles. During the development of these models the students will learn how to develop a flowsheet using unit operations and how to analyze the effects of various feedstocks and operating conditions on the final products, using pyrolysis and gasification as examples. The students will compare the impact of using renewable resources versus fossil fuels, such as coal or natural gas. During the Year 2 the participant students will use experimental tools to realize the results of Year 1 in practice. The students will use two of the most efficient resources found in Year 1 and will perform Thermogravimentric Analysis (TGA) and Pyrolysis Gas Chromatography Analysis (PyGC) experiments. Using these relatively simple experimental tools, the undergraduate students will have hands-on experience on the production of H2 and other fuels using carbon-based renewable resources. During the Year 3 of the REU sites the students will be involved in the catalyst synthesis. Following the results of Year 1 and 2 the students will transition from modeling and thermodynamic studies to catalytic studies and will learn how the various catalysts can affect the conversion and the selectivity of the reactions to H2.
Expected Outcomes: During this project students will learn how to apply fundamental knowledge of unit operations, thermodynamics and kinetics to understand how the renewable carbon resources (biomass) can be used to produce H2.
Project 1.2 – Enhance Biogas Production and System Resilience (Baikun Li)
Background: Anaerobic digestion (AD) has been recently highlighted as an environmentally friendly method to produce methane (CH4) as well as H2 from food, animal waste or wastewater. In this process, microorganisms decompose biomass in the absence of oxygen. Through AD, waste is recycled to produce H2 gas and liquid fermentation products that can be subsequently converted to methane or biogas. However, there are still many challenges related with AD.
Objectives: During this project the participant students will develop real-time monitoring and profiling methodologies to enhance biogas production and system resilience of AD systems treating organic wastes. AD systems have been widely used as bioresource recovery and biogas production in wastewater treatment plants, but notoriously suffer from problems such as over-acidification, biomass loss, poor resilience, and low biogas yield. In this project, students will deploy mm-sized electrode array (MEA) sensors that have been developed in Dr. Baikun Li’s group to a lab-scale continuous flow AD system (volume: 2L). Multiple pieces of five types of MEAs (temperature, redox potential (ORP), pH, conductivity and ammonium) will be installed along the depth and perimeters of AD systems to obtain high-fidelity profiles of the AD under different operational conditions (e.g. organic loading, retention time, mixing regime). The students will integrate the continuous on-line MEA sensor profiles with discrete off-line measurement (e.g. biomass concentration, volatile fatty acid, and biogas content) to gain a complete picture of the physiochemical and biochemical status of the AD. Especially, the students will grasp the concept of real-time high resolution profile and sensor data analysis visualization. Along with MEA technology, they will learn to develop data-driven models for system control and predict bioresource recovery and treatment efficiency under steady status or transient shocks.
Expected Outcomes: Throughout this project, students will learn the frontier system visualization technology and its importance in bioresource recovery from AD systems.
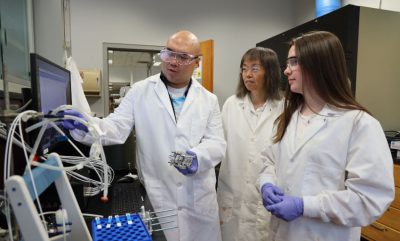
Project 1.3 – Development of Cost-effective Water Electrolyzer (Stoyan Bliznakov)
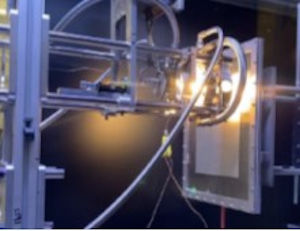
Background: H2 generation by water electrolysis is a low-temperature and highly efficient technology that has the potential to ensure large quantity H2 production without CO2 emission. However, the commercialization of this technology is hindered by the use of the expensive platinum group metals (PGMs) as catalysts for water splitting. At UConn we have developed a novel technology that allows to reduce the PGM catalyst loadings in the proton exchange membrane water electrolyzers (PEMWEs) by one order of magnitude. This innovative methodology is known as Reactive Spray Deposition Technology (RSDT) and allows to manufacture large scale membrane electrode assemblies (MEAs) with industrial significance. The RSDT is a flame-based method that allows one-step deposition of the catalysts directly on the PEM membrane.
Objectives: During Year 1 the participating students will be introduced to the field of water electrolysis by starting with overview of the literature. Simultaneously, they will be trained in using RSDT for deposition of Pt nanoparticles supported on carbon (Pt/C) directly on Nafion membranes. The focus of this research will be to optimize the conditions for deposition of the cathode catalyst layers. The students will fabricate the Pt/C catalyst layers and will characterize the deposits with Scanning Electron Microscopy (SEM) and Inductively Coupled Plasma (ICP). They will study the nanoparticles’ shape, morphology, composition, and particles size distribution by high-resolution Transmission Electron Microscopy (TEM). After optimizing the deposition parameters for the cathode catalyst layer fabrication, the REU students will assemble full MEAs by using standard anode catalyst layers and standard recombination layers (RL) and will evaluate their performance at operating conditions that are typical for an industrial electrolyzers. During Year 2 of the project the new REU students will start their research experience with a literature review in the field of PEMWEs, and then will focus on the development and optimization of the iridium anode catalyst layer. The REU students will follow the steps described above and will deposit the anode catalyst layers by the RSDT method. All deposition parameters will be optimized, and the samples will be characterized by the techniques described above. The REU students will fabricate MEAs with optimized anodes and their performance will be evaluated and compared to the state-of-the-art commercial MEAs. During Year 3, the REU students will focus on the optimization of the conditions for the deposition of the RL. The students will use the RSDT to fabricate a set of Pt RLs with various thicknesses, and then will characterize each sample. The optimized conditions will be used to fabricate RLs and MEAs with the best anode and cathode layers and their performance will be studied and compared to the MEAs reported in the literature.
Expected Outcomes: The REU students will be involved in cutting-edge research activities in the field of PEMWEs that is funded by the DOE, they will be trained to fabricate advanced MEAs and they will learn how to use the state-of-the-art techniques for materials characterization. The students will receive feedback directly from our industrial collaborator, Nel Hydrogen, who is a leader in production of PEMWEs.
Project 1.4 – Microscopical Insights into the World of Clean Energy (Jasna Jankovic)
Background: H2 generation devices (electrolyzers) and power generation devices (fuel cells) have very intriguing microstructure. From nanometer-sized catalyst particles, to millimeter-sized flow field plates – these micro/macroscopical features determine the efficiency and durability of the devices. 2D SEM and TEM have routinely been used to characterize materials in these devices, but with the advancement of microscopy 3D imaging is gaining a huge interest. The Thermo Fisher Scientific Center for Advanced Microscopy and Materials Analysis (CAMMA) at UConn offers cutting-edge microscopy facilities for investigation of materials for sustainable energy systems. Dr. Jankovic’s (member of CAMMA) research interest lies in the development and application of advanced imaging and spectroscopy techniques.
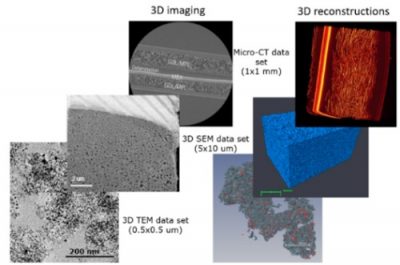
Objectives: In this project, students will be exposed to a range of microscopy methods from light microscopy, SEM and TEM to X-ray computed tomography (micro-CT). Especially interesting will be the application of 3D imaging and reconstruction of realistic 3D models. The students will learn about materials used in these devices, their properties and ways to characterize these components. The project will be organized in a similar manner each year and will be closely coordinated with other projects that involve materials characterization, such as Projects 1.1, 1.3 and 3.1.
Expected Outcomes: The students will learn about various microscopy techniques, will have hands-on experience with microscopy sample preparation and characterization, will be trained in data analysis and will learn about the components of fuel cells and electrolyzers. Dr Jankovic’s research is funded by DOE, NSF and industrial partners such as Giner Inc. (leader in the technology of electrolyzers) and Ford Motor company.
Project 1.5 – Design of an impedance measurement system for fuel cell/electrolyzer using a power converter (Sung Yeul Park & Jasna Jankovic)
Background: Batteries, electrolyzers, and fuel cells are electrochemical devices to store electricity, generate H2, and produce electricity, respectively. Electrochemical Impedance Spectroscopy (EIS) has been applied to these devices to analyze their characteristics at the microscale level in the lab using specialized instruments. Due to low cost of high-performing processors, online EIS functionality can be embedded in power converters, which not only provide power transfer between electrochemical devices and power sources/loads, but also examine the conditions of electrochemical devices. Dr. Park’s research interest lies in the field of renewable energy power conditioning systems through power electronics and has active research projects on real time impedance utilization in the Li-Ion battery applications.
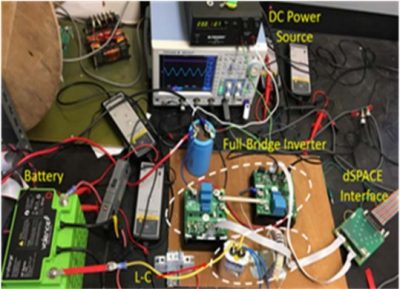
Objective: This project aims to create a prototype for impedance measurement of fuel cell/electrolyzer systems by adding AC ripple current on top of operating DS current and measuring AC voltage of the fuel cell membrane or electrolyzer stacks using the power converter. The Figure shows a battery testbed used similarly for AC current injection. During Year 1 the student will focus on building a simulation model for power converter and fuel cell/electrolyzer as electrical equivalent circuit approach, and on designing circuit and test bed for prototype system. In Year 2 the student will focus on building a small scale online EIS embedded power converter. In Year 3 the student will focus on validation of the performance and parameter variations and on reconfiguration of existing power supply.
Expected Outcomes: By the end of each task, students will learn: 1) the components of electro-chemical devices, 2) how the electrochemical characteristics can be modeled as electrical equivalent circuit models, 3) the power converter’s role and operation, 4) how the power converter can measure impedance of electrochemical device, and 5) how to interpret impedance data. The outcomes of this project will provide preliminary data for the advanced prognosis tool for fuel cell and electrolyzer plants.